What is Impedance?
Impedance is an essential electrical property that describes the opposition a circuit presents to the flow of alternating current (AC) or any time-varying signal. It is a complex quantity that combines resistance and reactance, taking into account the effects of both the magnitude and phase of the signal. In simple terms, impedance measures how much a circuit resists or impedes the flow of current when an AC voltage is applied.
The concept of impedance is particularly relevant in the context of PCBs, where signals propagate through conductive traces and interact with various components. Understanding and controlling impedance on PCBs is crucial for maintaining signal integrity, minimizing reflections, and ensuring proper functioning of the electronic system.
Impedance Formula
Impedance is represented by the symbol “Z” and is measured in ohms (Ω). The impedance of a circuit is given by the following formula:
Z = R + jX
where:
– Z is the impedance in ohms (Ω)
– R is the resistance in ohms (Ω)
– X is the reactance in ohms (Ω)
– j is the imaginary unit (√-1)
The resistance (R) represents the opposition to the flow of current due to the inherent properties of the material, while the reactance (X) represents the opposition to the flow of current due to the presence of capacitive or inductive elements in the circuit.
Impedance vs. Resistance
It is important to distinguish between impedance and resistance. While resistance is a measure of the opposition to the flow of direct current (DC) in a circuit, impedance encompasses both resistance and reactance, taking into account the effects of AC signals.
In a purely resistive circuit, the impedance is equal to the resistance. However, in circuits with capacitive or inductive elements, the impedance varies with the frequency of the applied signal. This frequency-dependent behavior of impedance is a key consideration in PCB design, especially when dealing with high-speed signals.
Types of Impedance
There are several types of impedance that are relevant in the context of PCBs. Understanding these different types of impedance is crucial for designing and analyzing PCBs effectively.
Characteristic Impedance
Characteristic impedance, denoted as Z0, is a fundamental property of a transmission line, such as a PCB trace. It represents the ratio of the voltage to the current at any point along the transmission line when the line is infinitely long or properly terminated to prevent reflections.
The characteristic impedance of a PCB trace depends on various factors, including the trace width, trace thickness, dielectric constant of the PCB material, and the height of the trace above the ground plane. The characteristic impedance is typically expressed in ohms (Ω) and is an important parameter to consider when designing high-speed PCBs.
Common values for characteristic impedance in PCB design include:
Characteristic Impedance (Ω) | Application |
---|---|
50 | Coaxial cables, RF systems |
75 | Video systems, cable television |
90-120 | High-speed digital systems, Ethernet |
100 | Differential signaling (e.g., LVDS, USB) |
Matching the characteristic impedance of PCB traces to the impedance of the source and load is essential for minimizing reflections and ensuring efficient power transfer.
Input Impedance
Input impedance refers to the impedance seen by a signal source when it is connected to the input of a circuit or device. It is the equivalent impedance that the source encounters when driving the input of the circuit.
In the context of PCBs, input impedance is an important consideration when interfacing different components or modules. Mismatches between the source impedance and the input impedance can lead to signal reflections, distortion, and power loss.
To ensure proper signal integrity, it is crucial to match the output impedance of the driving circuit with the input impedance of the receiving circuit. This can be achieved through proper termination techniques, such as series or parallel termination resistors.
Output Impedance
Output impedance, on the other hand, refers to the impedance seen by a load when it is connected to the output of a circuit or device. It is the equivalent impedance that the load encounters when being driven by the output of the circuit.
Similar to input impedance, matching the output impedance of a driving circuit with the impedance of the load is important for maximizing power transfer and minimizing signal reflections. Mismatches between the output impedance and the load impedance can result in signal degradation and reduced performance.
PCB designers often use impedance matching techniques, such as source termination or series termination, to ensure proper matching between the output impedance of a driving circuit and the impedance of the load.
Importance of Impedance Control on PCBs
Controlling impedance on PCBs is crucial for several reasons. Let’s explore the key aspects that make impedance control essential in PCB design.
Signal Integrity
One of the primary reasons for controlling impedance on PCBs is to maintain signal integrity. When a signal travels along a PCB trace, it can encounter impedance mismatches, which cause reflections and distortions in the signal waveform. These reflections can lead to signal degradation, crosstalk, and even false triggering of digital circuits.
By carefully designing PCB traces with controlled impedance and implementing proper termination techniques, designers can minimize reflections and ensure that signals maintain their intended shape and timing. This is particularly important for high-speed digital signals, where even small reflections can cause significant issues.
Electromagnetic Compatibility (EMC)
Controlling impedance on PCBs also plays a vital role in achieving electromagnetic compatibility (EMC). EMC refers to the ability of electronic devices to operate properly in the presence of electromagnetic interference (EMI) and to not emit excessive EMI that can affect other devices.
Impedance mismatches on PCBs can lead to the radiation of electromagnetic energy, causing EMI issues. By designing PCB traces with controlled impedance and implementing appropriate shielding and grounding techniques, designers can minimize EMI radiation and ensure compliance with EMC regulations.
Power Integrity
Impedance control is not limited to signal traces; it also applies to power distribution networks (PDNs) on PCBs. The impedance of the PDN can have a significant impact on the power integrity of the system.
A low-impedance PDN is desirable to provide a stable and clean power supply to the components on the PCB. High-impedance power traces can lead to voltage fluctuations, noise, and power supply ripple, which can adversely affect the performance and reliability of the system.
Designers use various techniques, such as proper decoupling, power plane design, and the use of low-impedance capacitors, to control the impedance of the PDN and ensure power integrity on PCBs.
High-Speed Design Considerations
As electronic systems continue to push the boundaries of speed and bandwidth, impedance control becomes increasingly critical. High-speed digital interfaces, such as USB, PCIe, and HDMI, have strict impedance requirements to ensure proper signal transmission and reception.
In high-speed PCB designs, designers must carefully consider factors such as trace geometry, dielectric materials, and layer stackup to achieve the desired impedance characteristics. They may also need to employ advanced techniques, such as differential signaling and impedance matching, to maintain signal integrity at high frequencies.
Failure to control impedance in high-speed designs can result in signal distortion, jitter, and bit errors, compromising the overall system performance and reliability.
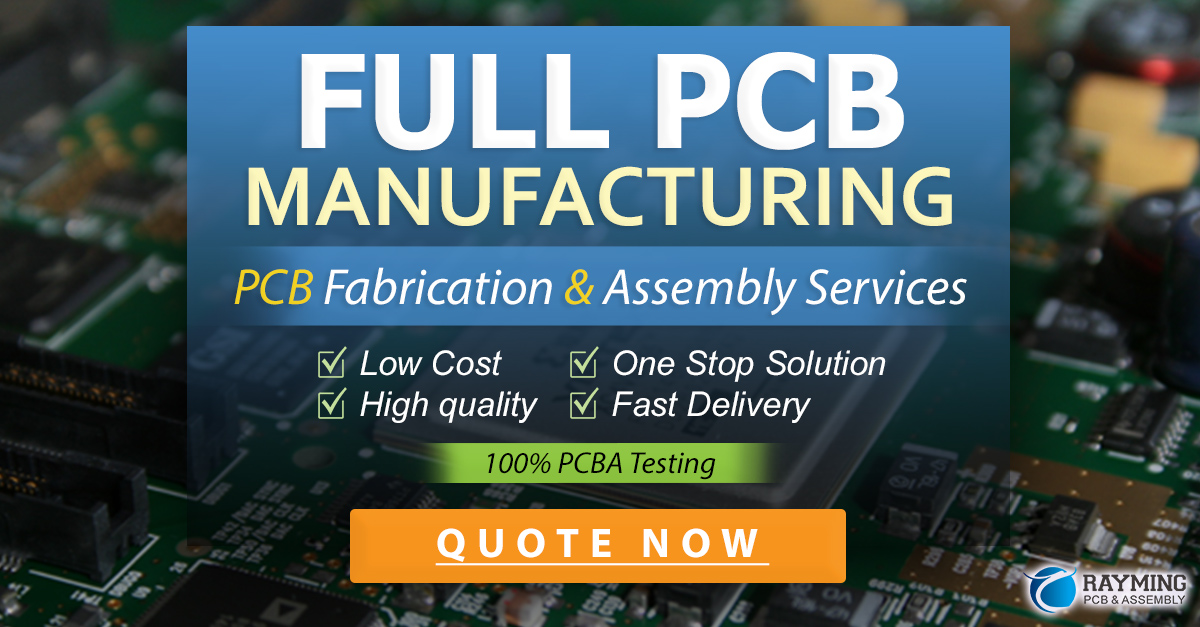
Methods for Controlling Impedance on PCBs
To achieve proper impedance control on PCBs, designers have several methods and techniques at their disposal. Let’s explore some of the common approaches used in PCB design.
Trace Geometry
One of the primary factors that influence the impedance of a PCB trace is its geometry. The width, thickness, and spacing of the traces play a crucial role in determining their characteristic impedance.
Designers use impedance calculators or simulation tools to determine the appropriate trace geometry for a given impedance value. By adjusting the trace width and thickness, they can achieve the desired characteristic impedance while considering manufacturing constraints and PCB layout requirements.
Dielectric Material Selection
The choice of dielectric material used in the PCB also affects the impedance of the traces. The dielectric constant (Dk) and dissipation factor (Df) of the material determine how the electromagnetic fields propagate through the PCB and influence the impedance.
Designers select dielectric materials with suitable Dk and Df values to achieve the desired impedance characteristics. Common dielectric materials used in PCB fabrication include FR-4, Rogers, and Isola materials, each with different properties and impedance control capabilities.
Layer Stackup Design
The layer stackup of a PCB refers to the arrangement of conductive layers and dielectric layers in the board. The layer stackup design plays a significant role in impedance control, as it determines the spacing between the traces and the reference planes.
Designers strategically place signal traces and their corresponding reference planes (ground or power) to maintain a consistent impedance throughout the PCB. They may also use techniques such as microstrip or stripline routing to achieve controlled impedance traces.
Termination Techniques
Proper termination is essential for controlling impedance and minimizing reflections on PCBs. Termination techniques involve adding resistors or other components at the end of a transmission line to match the impedance of the source or load.
Common termination techniques include:
- Series termination: A resistor is placed in series with the signal source to match the impedance of the trace.
- Parallel termination: A resistor is placed in parallel with the load to match the impedance of the trace.
- AC termination: Capacitors and resistors are used to provide a high-frequency termination while allowing DC bias.
Designers select the appropriate termination technique based on the specific requirements of the circuit, such as signal speed, power consumption, and available board space.
Impedance Matching Networks
In some cases, designers may need to use impedance matching networks to match the impedance between different sections of a PCB or between the PCB and external components. Impedance matching networks consist of passive components, such as resistors, capacitors, and inductors, arranged in a specific configuration to transform the impedance.
Common impedance matching networks include L-networks, Pi-networks, and T-networks. These networks are designed to provide the necessary impedance transformation while minimizing signal loss and reflections.
PCB Design Best Practices for Impedance Control
To ensure effective impedance control in PCB design, designers should follow certain best practices. Let’s explore some key guidelines for designing PCBs with controlled impedance.
Consistent Trace Width and Spacing
Maintaining consistent trace width and spacing is crucial for achieving uniform impedance throughout the PCB. Designers should adhere to the calculated trace geometry and avoid abrupt changes in trace width or spacing, as these can introduce impedance discontinuities and cause signal reflections.
Ground Plane Continuity
Ensuring continuity of the ground plane is essential for maintaining a low-impedance return path for signals. Designers should avoid splitting or interrupting the ground plane, as this can lead to impedance mismatches and signal integrity issues. If a ground plane must be discontinued, designers should use stitching vias or impedance-controlled bridges to maintain continuity.
Minimize Vias and Layer Transitions
Vias and layer transitions can introduce impedance discontinuities and cause signal reflections. Designers should minimize the use of vias and layer transitions in high-speed signal paths. When vias are necessary, they should be placed strategically to minimize their impact on impedance and signal integrity.
Length Matching and Delay Tuning
In high-speed designs, it is important to match the lengths of signal traces to ensure synchronous arrival of signals at their destinations. Designers should use length matching techniques, such as serpentine routing or delay lines, to equalize the propagation delays of signals.
Simulation and Verification
Simulating and verifying the impedance of PCB traces is an essential step in the design process. Designers should use electronic design automation (EDA) tools to perform impedance simulations and analyze the results. These simulations help identify potential impedance mismatches and allow designers to make necessary adjustments before finalizing the PCB layout.
Collaboration with PCB Fabricators
Working closely with PCB fabricators is crucial for ensuring the successful implementation of impedance control. Designers should communicate their impedance requirements clearly to the fabricator and provide detailed specifications, such as trace geometry, dielectric material, and layer stackup.
PCB fabricators have experience in manufacturing controlled impedance boards and can provide guidance on design rules, tolerances, and manufacturing capabilities. Collaborating with the fabricator throughout the design process can help avoid potential issues and ensure the PCB meets the desired impedance characteristics.
Frequently Asked Questions (FAQ)
-
What is the difference between impedance and resistance?
Impedance is a broader concept that encompasses both resistance and reactance. While resistance represents the opposition to the flow of direct current (DC), impedance takes into account the opposition to the flow of alternating current (AC) or any time-varying signal. Impedance is a complex quantity that includes both the magnitude and phase of the signal, whereas resistance is purely resistive and independent of frequency. -
Why is impedance matching important in PCB design?
Impedance matching is crucial in PCB design to ensure proper signal transmission and minimize reflections. When there is an impedance mismatch between the source, transmission line (PCB trace), and load, a portion of the signal energy is reflected back towards the source. These reflections can cause signal distortion, ringing, and other signal integrity issues. By matching the impedances of the source, trace, and load, designers can minimize reflections and ensure efficient power transfer, leading to better signal quality and system performance. -
How does the dielectric material affect the impedance of PCB traces?
The dielectric material used in the PCB plays a significant role in determining the impedance of the traces. The dielectric constant (Dk) of the material influences the speed of signal propagation and the characteristic impedance of the traces. A higher Dk value results in slower signal propagation and lower characteristic impedance, while a lower Dk value leads to faster signal propagation and higher characteristic impedance. The dissipation factor (Df) of the dielectric material also affects the impedance by introducing losses. Designers must carefully select the appropriate dielectric material based on the desired impedance characteristics and the specific requirements of the application. -
What are the common methods for achieving controlled impedance on PCBs?
There are several methods commonly used to achieve controlled impedance on PCBs: - Trace geometry: Adjusting the width, thickness, and spacing of the traces to achieve the desired characteristic impedance.
- Dielectric material selection: Choosing a dielectric material with suitable Dk and Df values to achieve the desired impedance.
- Layer stackup design: Strategically arranging the conductive and dielectric layers in the PCB to maintain consistent impedance.
- Termination techniques: Using series, parallel, or AC termination resistors to match the impedance of the source or load.
-
Impedance matching networks: Employing passive components, such as resistors, capacitors, and inductors, to transform the impedance between different sections of the PCB.
-
What are some best practices for designing PCBs with controlled impedance?
Some best practices for designing PCBs with controlled impedance include: - Maintaining consistent trace width and spacing throughout the PCB.
- Ensuring continuity of the ground plane and avoiding interruptions.
- Minimizing the use of vias and layer transitions in high-speed signal paths.
- Employing length matching and delay tuning techniques to synchronize signal arrival times.
- Performing impedance simulations and verifications using EDA tools.
- Collaborating closely with PCB fabricators to ensure the successful implementation of impedance control.
By following these best practices, designers can effectively control impedance on PCBs and achieve optimal signal integrity and system performance.
Conclusion
Impedance control is a critical aspect of PCB design that ensures signal integrity, minimizes reflections, and optimizes system performance. Understanding the concept of impedance, its various types, and its significance on PCBs is essential for designers working on high-speed and high-frequency applications.
By employing techniques such as trace geometry optimization, dielectric material selection, layer stackup design, and proper termination, designers can effectively control impedance on PCBs. Following best practices, such as maintaining consistent trace geometry, ensuring ground plane continuity, and collaborating with PCB fabricators, further enhances the success of impedance-controlled designs.
As electronic systems continue to push the boundaries of speed and performance, the importance of impedance control in PCB design will only continue to grow. By mastering the principles and techniques of impedance control, designers can create robust and reliable PCBs that meet the demanding requirements of modern electronics.
Leave a Reply