Introduction to RF Amplifiers
An RF (radio frequency) amplifier is an electronic device or circuit that increases the power of an RF signal. RF amplifiers are essential components in many wireless communication systems, including cellular networks, Wi-Fi, Bluetooth, and satellite communications. They are used to boost the signal strength of transmitted or received signals, compensating for losses in the transmission medium and ensuring that the signal reaches its intended destination with sufficient power.
RF amplifiers operate at high frequencies, typically in the range of megahertz (MHz) to gigahertz (GHz). Designing RF amplifiers requires careful consideration of various factors, such as gain, bandwidth, linearity, efficiency, and noise performance. This guide will provide a comprehensive overview of RF Amplifier Design, covering the fundamental concepts, design techniques, and practical applications.
Key Parameters in RF Amplifier Design
When designing an RF amplifier, several key parameters need to be considered to ensure optimal performance. These parameters include:
Gain
Gain is a measure of how much the amplifier increases the power of the input signal. It is usually expressed in decibels (dB) and can be calculated using the following formula:
Gain (dB) = 10 log₁₀ (Pout / Pin)
where Pout is the output power and Pin is the input power.
Bandwidth
Bandwidth refers to the range of frequencies over which the amplifier can operate effectively. It is determined by the upper and lower cutoff frequencies, which are the points where the gain of the amplifier drops by 3 dB from its maximum value.
Linearity
Linearity is a measure of how well the amplifier’s output signal remains proportional to its input signal. Non-linearities in the amplifier can cause distortion in the output signal, which can degrade the quality of the communication. Linearity is often quantified using metrics such as the 1 dB compression point (P1dB) and the third-order intercept point (IP3).
Efficiency
Efficiency is a measure of how much of the DC power supplied to the amplifier is converted into useful RF output power. It is usually expressed as a percentage and can be calculated using the following formula:
Efficiency (%) = (Pout / PDC) × 100
where Pout is the RF output power and PDC is the DC power supplied to the amplifier.
Noise Figure
The noise figure is a measure of how much noise the amplifier adds to the signal. It is defined as the ratio of the signal-to-noise ratio (SNR) at the input of the amplifier to the SNR at the output. A lower noise figure indicates better noise performance.
RF Amplifier Topologies
There are several common RF amplifier topologies, each with its own advantages and disadvantages. The choice of topology depends on the specific requirements of the application, such as gain, bandwidth, linearity, and efficiency. Some of the most common RF amplifier topologies include:
Class A Amplifiers
Class A amplifiers are the most linear type of amplifier, but they are also the least efficient. They operate with a constant DC bias current, and the output signal is a scaled version of the input signal. Class A amplifiers are often used in applications where linearity is critical, such as in low-noise amplifiers (LNAs) and driver amplifiers.
Class B Amplifiers
Class B amplifiers are more efficient than Class A amplifiers but have higher distortion. They use two complementary transistors, each conducting for half of the input signal cycle. The DC bias current is zero when there is no input signal, which improves efficiency. However, the transition between the two transistors can cause crossover distortion.
Class AB Amplifiers
Class AB amplifiers are a compromise between Class A and Class B amplifiers. They operate with a small amount of DC bias current, which reduces crossover distortion compared to Class B amplifiers while maintaining higher efficiency than Class A amplifiers. Class AB amplifiers are commonly used in power amplifiers for wireless communication systems.
Class C Amplifiers
Class C amplifiers are the most efficient type of amplifier but have the highest distortion. They operate with a negative DC bias, which means that the transistor only conducts for a small portion of the input signal cycle. Class C amplifiers are often used in high-power applications where efficiency is critical, such as in radar systems and particle accelerators.
Class D Amplifiers
Class D amplifiers, also known as switching amplifiers, use pulse-width modulation (PWM) to convert the input signal into a series of pulses. These pulses are then filtered to reconstruct the amplified output signal. Class D amplifiers are highly efficient but have limited bandwidth and can introduce switching noise.
Class E Amplifiers
Class E amplifiers are a type of switching amplifier that uses a single transistor and a tuned load network. They are designed to minimize switching losses and can achieve very high efficiency, often exceeding 90%. Class E amplifiers are commonly used in high-frequency applications, such as in RF power amplifiers for wireless communication systems.
Amplifier Class | Linearity | Efficiency | Applications |
---|---|---|---|
Class A | High | Low (< 50%) | LNAs, driver amplifiers |
Class B | Moderate | Moderate (≈ 78.5%) | Audio amplifiers |
Class AB | Moderate to High | Moderate to High (50-78.5%) | Power amplifiers for wireless communication |
Class C | Low | High (> 78.5%) | High-power applications (radar, particle accelerators) |
Class D | Moderate | High (> 90%) | Audio amplifiers, low-frequency power amplifiers |
Class E | Low to Moderate | Very High (> 90%) | RF power amplifiers for wireless communication |
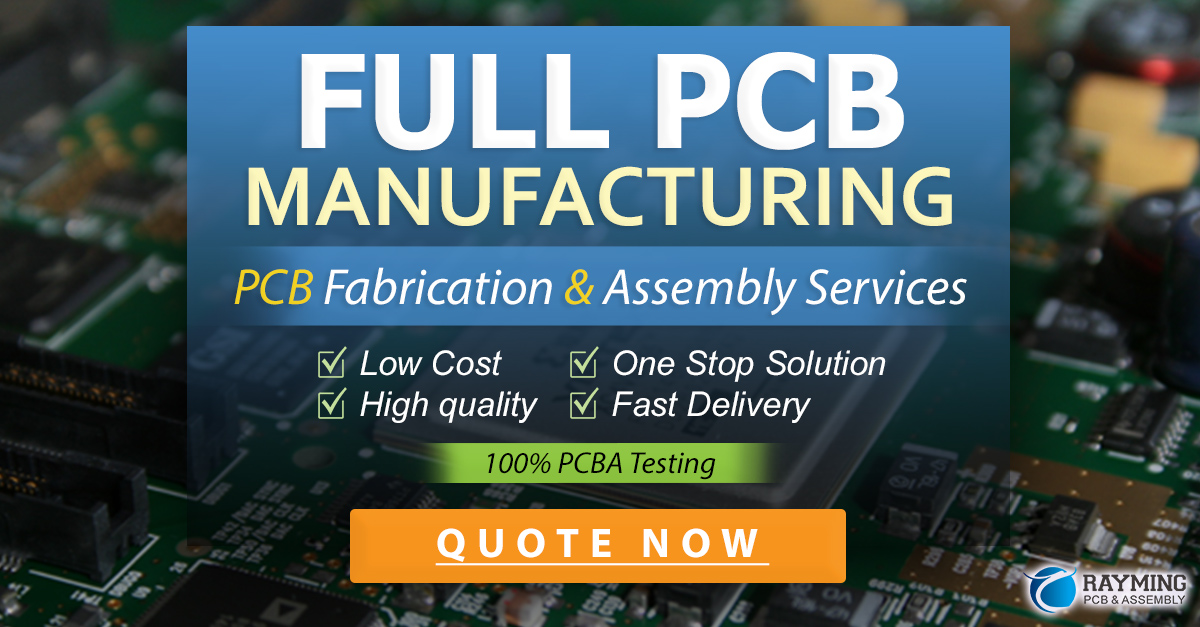
RF Amplifier Design Techniques
Designing high-performance RF amplifiers requires the use of various techniques to optimize gain, bandwidth, linearity, efficiency, and noise performance. Some of the most common RF amplifier design techniques include:
Impedance Matching
Impedance matching is the process of designing the input and output networks of the amplifier to ensure maximum power transfer and minimize reflections. This is typically achieved using passive components such as inductors, capacitors, and transmission lines. Proper impedance matching is critical for achieving optimal gain, bandwidth, and efficiency.
Feedback
Feedback is a technique used to improve the linearity and stability of RF amplifiers. There are two main types of feedback: negative feedback and positive feedback. Negative feedback reduces the gain of the amplifier but improves its linearity and bandwidth. Positive feedback increases the gain of the amplifier but can cause instability if not carefully designed.
Biasing
Biasing is the process of setting the DC operating point of the amplifier’s active devices, such as transistors. Proper biasing is essential for achieving the desired gain, linearity, and efficiency. Different biasing techniques can be used depending on the amplifier topology and the specific requirements of the application.
Linearization Techniques
Linearization techniques are used to improve the linearity of RF amplifiers, particularly in high-power applications where non-linearities can cause significant distortion. Some common linearization techniques include:
- Predistortion: A technique where the input signal is intentionally distorted in a way that cancels out the non-linearities of the amplifier.
- Feedforward: A technique where the output of the amplifier is compared to a delayed version of the input signal, and the error signal is used to cancel out the distortion.
- Envelope tracking: A technique where the supply voltage of the amplifier is dynamically adjusted to match the envelope of the input signal, improving efficiency and linearity.
Thermal Management
Thermal management is an important consideration in RF amplifier design, particularly in high-power applications. Proper heat dissipation is essential for ensuring the reliability and long-term performance of the amplifier. This can be achieved through the use of heat sinks, cooling fans, and other thermal management techniques.
RF Amplifier Applications
RF amplifiers are used in a wide range of applications, from wireless communication systems to radar and scientific instruments. Some of the most common applications include:
Wireless Communication Systems
RF amplifiers are essential components in wireless communication systems, such as cellular networks, Wi-Fi, and Bluetooth. They are used in both the transmitter and receiver chains to boost the signal strength and compensate for losses in the transmission medium. RF power amplifiers are particularly important in the transmitter chain, as they are responsible for generating the high-power RF signal that is transmitted by the antenna.
Radar Systems
RF amplifiers are used in radar systems to generate high-power RF pulses that are transmitted by the antenna. They are also used in the receiver chain to amplify the weak reflected signals from targets. High-power RF amplifiers, such as traveling wave tube amplifiers (TWTAs) and solid-state power amplifiers (SSPAs), are commonly used in radar applications.
Satellite Communications
RF amplifiers are used in satellite communication systems to amplify the signals transmitted from the ground to the satellite and vice versa. They are also used within the satellite itself to amplify the signals before they are transmitted back to Earth. Traveling wave tube amplifiers (TWTAs) are commonly used in satellite applications due to their high efficiency and reliability.
Scientific Instruments
RF amplifiers are used in various scientific instruments, such as particle accelerators, nuclear magnetic resonance (NMR) spectrometers, and radio telescopes. In particle accelerators, high-power RF amplifiers are used to generate the electromagnetic fields that accelerate the particles. In NMR spectrometers, RF amplifiers are used to excite the sample and detect the resulting NMR signals. In radio telescopes, low-noise amplifiers (LNAs) are used to amplify the weak signals from distant astronomical sources.
Application | Frequency Range | Power Level | Common Amplifier Types |
---|---|---|---|
Cellular Networks | 700 MHz – 3.5 GHz | 1 W – 100 W | Class AB, Doherty |
Wi-Fi | 2.4 GHz, 5 GHz | 100 mW – 1 W | Class AB, Class E |
Bluetooth | 2.4 GHz | 1 mW – 100 mW | Class AB, Class E |
Radar | 1 GHz – 40 GHz | 1 kW – 1 MW | TWTA, SSPA |
Satellite Communications | 1 GHz – 40 GHz | 1 W – 1 kW | TWTA, Class AB |
Particle Accelerators | 100 MHz – 3 GHz | 1 kW – 1 MW | Klystron, Solid-State |
NMR Spectrometers | 300 MHz – 1 GHz | 100 W – 1 kW | Class A, Class AB |
Radio Telescopes | 100 MHz – 100 GHz | 1 μW – 1 mW | LNA, Cryogenic Amplifiers |
Frequently Asked Questions (FAQ)
1. What is the difference between a low-noise amplifier (LNA) and a power amplifier (PA)?
A low-noise amplifier (LNA) is designed to amplify weak signals while adding minimal noise, making it ideal for use in receiver chains. A power amplifier (PA) is designed to deliver high output power to drive antennas or other loads, making it suitable for use in transmitter chains. LNAs prioritize low noise figure and high gain, while PAs prioritize high output power and efficiency.
2. What is the purpose of impedance matching in RF amplifier design?
Impedance matching is used to ensure maximum power transfer between the source, the amplifier, and the load. By matching the impedances of these components, reflections and power loss are minimized, resulting in improved gain, bandwidth, and efficiency.
3. What are the main differences between linear and non-linear RF amplifiers?
Linear RF amplifiers, such as Class A and Class AB, provide an output signal that is a scaled version of the input signal, with minimal distortion. Non-linear RF amplifiers, such as Class C and Class E, have higher efficiency but introduce more distortion in the output signal. The choice between linear and non-linear amplifiers depends on the specific requirements of the application, such as linearity, efficiency, and bandwidth.
4. What is the role of biasing in RF amplifier design?
Biasing is the process of setting the DC operating point of the amplifier’s active devices, such as transistors. Proper biasing ensures that the amplifier operates in the desired region (e.g., Class A, Class AB, or Class C) and achieves the required gain, linearity, and efficiency. Biasing also helps to stabilize the amplifier’s performance over temperature variations and other environmental factors.
5. What are some common challenges in designing high-power RF amplifiers?
Designing high-power RF amplifiers presents several challenges, including managing heat dissipation, ensuring device reliability, and maintaining linearity at high output powers. Other challenges include minimizing power supply noise, ensuring stable operation under varying load conditions, and achieving high efficiency while meeting the required bandwidth and linearity specifications. Advanced techniques such as dynamic load modulation, envelope tracking, and digital predistortion can be used to address these challenges and optimize the performance of high-power RF amplifiers.
Conclusion
RF amplifier design is a critical aspect of many wireless communication systems and other applications that rely on high-frequency signals. Understanding the key parameters, topologies, and design techniques is essential for developing high-performance RF amplifiers that meet the specific requirements of each application.
This guide has provided a comprehensive overview of RF amplifier design, covering the fundamental concepts, common amplifier classes, design techniques, and practical applications. By considering factors such as gain, bandwidth, linearity, efficiency, and noise performance, engineers can design RF amplifiers that are optimized for their intended use cases.
As wireless communication systems continue to evolve and new applications emerge, the demand for advanced RF amplifier designs will only continue to grow. By staying up-to-date with the latest techniques and technologies, RF engineers can contribute to the development of faster, more reliable, and more efficient wireless systems that connect people and devices across the globe.
Leave a Reply