Introduction to EMF scanning
Electromagnetic field (EMF) scanning is a cutting-edge technology that has revolutionized the way we detect and measure electromagnetic fields in various environments. This non-invasive technique allows for rapid, accurate, and high-resolution mapping of electromagnetic fields, enabling researchers, engineers, and technicians to identify potential sources of interference, optimize electronic devices, and ensure compliance with safety regulations.
What is an Electromagnetic Field?
An electromagnetic field is a physical field produced by electrically charged objects or particles in motion. It consists of both electric and magnetic components that oscillate perpendicular to each other and propagate through space at the speed of light. Electromagnetic fields can be classified into two main categories:
-
Static fields: These fields do not vary with time and are produced by stationary charges or permanent magnets. Examples include the Earth’s magnetic field and the fields generated by DC power lines.
-
Time-varying fields: These fields change with time and are produced by alternating currents (AC) or accelerating charges. Examples include the fields generated by radio waves, microwaves, and visible light.
Importance of EMF Scanning
EMF scanning plays a crucial role in various industries and applications, such as:
-
Electromagnetic Compatibility (EMC) testing: EMF scanning helps ensure that electronic devices and systems do not interfere with each other or with other equipment in their vicinity. This is particularly important in industries such as telecommunications, automotive, and aerospace, where electromagnetic interference can lead to system failures or safety hazards.
-
Wireless communications: EMF scanning is used to optimize the performance of wireless communication systems, such as cellular networks, Wi-Fi, and Bluetooth. By mapping the electromagnetic fields generated by these systems, engineers can identify areas of weak signal coverage, minimize interference, and improve overall network efficiency.
-
Medical applications: EMF scanning is employed in various medical imaging techniques, such as Magnetic Resonance Imaging (MRI) and Magnetoencephalography (MEG). These techniques rely on the interaction between electromagnetic fields and biological tissues to generate detailed images of the human body or to study brain activity.
-
Scientific research: EMF scanning is a valuable tool for studying the properties and behavior of electromagnetic fields in various contexts, such as plasma physics, astrophysics, and materials science. By mapping the electromagnetic fields in these systems, researchers can gain insights into fundamental physical processes and develop new technologies.
Principles of EMF Scanning
EMF scanning relies on several key principles and techniques to accurately measure and map electromagnetic fields. These include:
Electromagnetic Field Sensors
At the heart of EMF scanning systems are specialized sensors that can detect and measure electromagnetic fields. These sensors come in various types, each with its own advantages and limitations:
-
Hall Effect Sensors: These sensors utilize the Hall effect, which is the production of a voltage difference across an electrical conductor when a magnetic field is applied perpendicular to the current flow. Hall effect sensors are commonly used for measuring static magnetic fields and low-frequency alternating fields.
-
Inductive sensors: Also known as search coils, these sensors consist of a coil of wire that generates an induced voltage when exposed to a changing magnetic field. Inductive sensors are well-suited for measuring time-varying magnetic fields, such as those produced by AC power lines or radio waves.
-
Antennas: Antennas are used to detect and measure high-frequency electromagnetic fields, such as those in the microwave and millimeter-wave ranges. Various types of antennas, such as dipoles, monopoles, and horn antennas, are employed depending on the frequency range and application.
-
Optically pumped magnetometers: These highly sensitive sensors use the interaction between light and atomic vapors to measure extremely weak magnetic fields. They are commonly used in applications such as geophysical surveys and medical imaging.
Scanning Techniques
EMF scanning systems employ various techniques to map electromagnetic fields in a given environment. These techniques can be broadly classified into two categories:
-
Point-by-point scanning: In this approach, the EMF sensor is moved manually or by a motorized stage to different positions within the scanning area. At each position, the sensor measures the local electromagnetic field, and the data is recorded. This technique is simple and versatile but can be time-consuming for large scanning areas.
-
Array scanning: This technique involves using an array of EMF sensors that can simultaneously measure the electromagnetic field at multiple points. Array scanning allows for faster data acquisition and higher spatial resolution compared to point-by-point scanning. However, it requires more complex hardware and signal processing algorithms.
Data Processing and Visualization
Once the electromagnetic field data has been acquired, it needs to be processed and visualized to extract meaningful information. This typically involves the following steps:
-
Calibration: The raw sensor data is calibrated to account for any systematic errors or variations in the sensor response. This ensures that the measured field values are accurate and consistent across different sensors and scanning sessions.
-
Filtering: The data is filtered to remove any unwanted noise or artifacts that may have been introduced during the scanning process. Various digital signal processing techniques, such as low-pass, high-pass, or band-pass filtering, can be employed depending on the nature of the noise and the desired signal.
-
Interpolation: In order to generate a continuous field map from discrete sensor measurements, interpolation algorithms are used to estimate the field values between the sampled points. Common interpolation methods include linear, cubic, and kriging interpolation.
-
Visualization: The processed data is then visualized using various graphical techniques, such as color maps, contour plots, or 3D surface plots. These visualizations help users to easily interpret the spatial distribution and intensity of the electromagnetic fields.
Applications of EMF Scanning
EMF scanning has found numerous applications across various industries and research fields. Some of the most notable applications include:
Electromagnetic Compatibility Testing
Electromagnetic compatibility (EMC) testing is a critical process in the development and certification of electronic devices and systems. It ensures that these devices can operate reliably in their intended electromagnetic environment without causing or suffering from excessive electromagnetic interference.
EMF scanning plays a crucial role in EMC testing by allowing engineers to map the electromagnetic fields generated by a device under test (DUT) and to identify potential sources of interference. By visualizing the field distribution around the DUT, engineers can pinpoint areas of high field intensity, locate leaks or gaps in electromagnetic shielding, and optimize the device layout to minimize emissions.
Moreover, EMF scanning can be used to assess the susceptibility of a DUT to external electromagnetic fields. By exposing the DUT to controlled electromagnetic fields and monitoring its performance, engineers can determine the device’s immunity to interference and develop appropriate countermeasures.
Wireless Network Optimization
The proliferation of wireless communication technologies, such as cellular networks, Wi-Fi, and Bluetooth, has led to an increasing demand for efficient and reliable wireless networks. EMF scanning is a valuable tool for optimizing the performance of these networks by mapping the electromagnetic fields generated by the network infrastructure and user devices.
By scanning the electromagnetic fields in a given environment, network engineers can identify areas of weak signal coverage, high interference, or excessive power consumption. This information can then be used to optimize the placement and configuration of network nodes, such as base stations, access points, or repeaters, to ensure uniform coverage and minimize interference.
EMF scanning can also help in the planning and deployment of new wireless networks. By simulating the electromagnetic field distribution based on the proposed network layout and environmental factors, engineers can predict the network performance and make necessary adjustments before the actual installation.
Medical Imaging and Diagnostics
EMF scanning has found significant applications in the medical field, particularly in imaging and diagnostic techniques that rely on the interaction between electromagnetic fields and biological tissues.
Magnetic Resonance Imaging (MRI) is a prime example of how EMF scanning is used in medical imaging. MRI scanners employ powerful magnetic fields and radio waves to generate detailed images of the human body’s internal structures. By manipulating the magnetic fields and measuring the resulting electromagnetic signals from the body tissues, MRI scanners can produce high-resolution images of organs, soft tissues, and bones, aiding in the diagnosis and monitoring of various medical conditions.
Another medical application of EMF scanning is Magnetoencephalography (MEG), a technique used to study brain activity. MEG scanners measure the weak magnetic fields generated by the electrical currents in the brain, allowing researchers to map the spatial and temporal patterns of neural activity. This information can be used to understand brain function, diagnose neurological disorders, and guide surgical interventions.
Scientific Research
EMF scanning is a fundamental tool in many areas of scientific research, enabling researchers to study the properties and behavior of electromagnetic fields in various physical systems.
In plasma physics, EMF scanning is used to investigate the complex electromagnetic interactions in plasmas, such as those found in fusion reactors, space plasmas, or laboratory experiments. By mapping the electromagnetic fields in these systems, researchers can gain insights into the underlying physical processes, such as plasma instabilities, wave-particle interactions, and magnetic reconnection.
In astrophysics, EMF scanning is employed to study the electromagnetic fields in celestial objects, such as stars, galaxies, and cosmic magnetic fields. By measuring the polarization and spectrum of the electromagnetic radiation from these objects, researchers can infer the strength and orientation of the magnetic fields, as well as the properties of the emitting particles.
In materials science, EMF scanning is used to characterize the electromagnetic properties of various materials, such as semiconductors, superconductors, and metamaterials. By measuring the response of these materials to applied electromagnetic fields, researchers can determine their electrical conductivity, magnetic permeability, and dielectric permittivity, which are essential for developing new electronic and photonic devices.
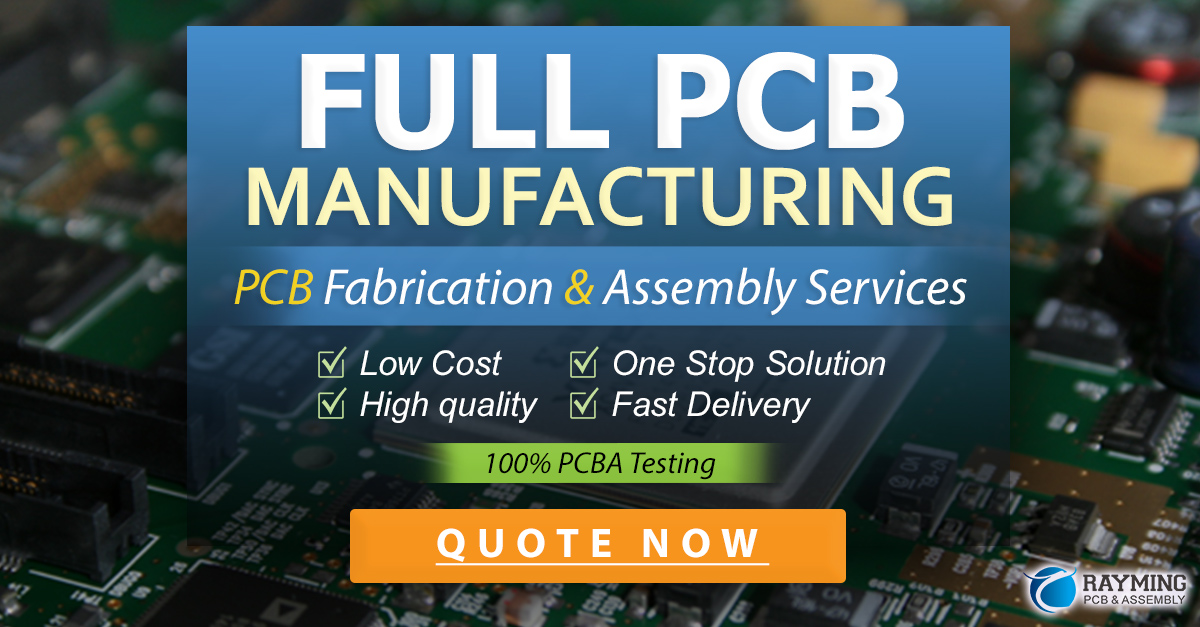
Advances in EMF Scanning Technology
The field of EMF scanning is continuously evolving, driven by advances in sensor technology, signal processing algorithms, and computational capabilities. Some of the recent developments in EMF scanning technology include:
High-Resolution Scanning
The spatial resolution of EMF scanning systems has been steadily improving over the years, thanks to the development of more sensitive and compact sensors, as well as advanced scanning techniques. High-resolution scanning allows for the mapping of electromagnetic fields with sub-millimeter accuracy, enabling researchers to study the fine-scale structure of electromagnetic phenomena and to optimize electronic devices with unprecedented precision.
One example of high-resolution EMF scanning is near-field scanning optical microscopy (NSOM), which combines the principles of optical microscopy and scanning probe microscopy to map the electromagnetic fields of nanoscale structures. NSOM uses a small aperture or a sharp tip to confine the light to a sub-wavelength spot, allowing for the imaging of electromagnetic fields with a resolution far beyond the diffraction limit.
Real-Time Scanning
Traditional EMF scanning techniques often involve a time-consuming process of data acquisition, processing, and visualization, limiting their usefulness in dynamic or fast-changing electromagnetic environments. However, recent advances in hardware and software have enabled the development of real-time EMF scanning systems that can acquire, process, and display electromagnetic field data in near real-time.
Real-time EMF scanning is particularly valuable in applications such as EMC testing, where the ability to quickly identify and locate sources of electromagnetic interference is crucial. By providing immediate feedback on the electromagnetic field distribution, real-time scanning allows engineers to make on-the-fly adjustments to the device under test, streamlining the debugging and optimization process.
Real-time scanning is also beneficial in medical applications, such as MRI-guided interventions, where the ability to continuously monitor the electromagnetic fields in the patient’s body can help ensure the safety and accuracy of the procedure.
Multi-Physics Scanning
Another emerging trend in EMF scanning is the integration of multiple physical sensing modalities to provide a more comprehensive understanding of the electromagnetic environment. Multi-physics scanning combines EMF scanning with other sensing techniques, such as thermal imaging, acoustic sensing, or particle detection, to correlate the electromagnetic field distribution with other physical phenomena.
For example, in the field of plasma physics, multi-physics scanning can be used to simultaneously map the electromagnetic fields, temperature, and particle fluxes in a plasma, providing insights into the complex interactions between these different physical processes. Similarly, in medical applications, multi-physics scanning can combine EMF scanning with physiological sensing, such as electroencephalography (EEG) or electrocardiography (ECG), to study the effects of electromagnetic fields on the human body.
Artificial Intelligence and Machine Learning
The increasing complexity and volume of data generated by EMF scanning systems have created a need for advanced data analysis and interpretation tools. Artificial intelligence (AI) and machine learning (ML) techniques are increasingly being applied to EMF scanning data to extract meaningful insights and automate various aspects of the scanning process.
ML algorithms can be trained on large datasets of EMF scanning results to recognize patterns, classify electromagnetic field distributions, and predict the behavior of electromagnetic systems. This can help researchers and engineers to quickly identify potential problems, optimize device designs, and develop new applications for EMF scanning technology.
AI-based techniques, such as deep learning and computer vision, can also be used to automate the processing and visualization of EMF scanning data. By learning to segment, label, and interpret electromagnetic field images, AI algorithms can significantly reduce the time and effort required for manual data analysis, allowing users to focus on higher-level tasks and decision-making.
Frequently Asked Questions (FAQ)
1. What is the difference between static and time-varying electromagnetic fields?
Static electromagnetic fields are those that do not change with time, such as the fields produced by permanent magnets or DC currents. In contrast, time-varying electromagnetic fields are those that oscillate or change over time, such as the fields generated by alternating currents (AC) or electromagnetic waves.
2. How does EMF scanning differ from other electromagnetic measurement techniques?
EMF scanning is distinguished from other electromagnetic measurement techniques by its ability to provide a spatial map of the electromagnetic field distribution in a given environment. While other techniques, such as spectrum analyzers or oscilloscopes, can measure the frequency and amplitude of electromagnetic signals at a single point, EMF scanning allows for the visualization of the field intensity and orientation across a larger area or volume.
3. What are the key components of an EMF scanning system?
A typical EMF scanning system consists of three main components:
- Electromagnetic field sensors, such as Hall effect sensors, inductive sensors, or antennas, which detect and measure the local electromagnetic field.
- A scanning mechanism, such as a motorized stage or a Robotic Arm, which moves the sensors to different positions within the scanning area.
- A data acquisition and processing unit, which collects the sensor data, performs necessary signal conditioning and analysis, and generates the final field map.
4. What are some of the challenges in EMF scanning?
Some of the main challenges in EMF scanning include:
- Ensuring the accuracy and repeatability of the sensor measurements, particularly in the presence of noise, interference, or environmental factors.
- Achieving high spatial resolution and fast scanning speeds, especially for large or complex scanning volumes.
- Developing efficient data processing and visualization algorithms that can handle the large amounts of data generated by high-resolution scanning.
- Integrating EMF scanning with other physical sensing modalities to provide a more comprehensive understanding of the electromagnetic environment.
5. What are some of the future trends in EMF scanning technology?
Some of the key trends shaping the future of EMF scanning technology include:
- The development of more sensitive, compact, and affordable electromagnetic field sensors, enabling the widespread adoption of EMF scanning in various industries and applications.
- The integration of artificial intelligence and machine learning techniques to automate data analysis, pattern recognition, and decision-making in EMF scanning systems.
- The increasing use of multi-physics scanning approaches that combine EMF scanning with other sensing modalities, such as thermal imaging or acoustic sensing, to provide a more holistic view of the electromagnetic environment.
- The development of real-time and in-situ EMF scanning systems that can provide continuous monitoring and feedback in dynamic or safety-critical applications.
Conclusion
Electromagnetic field high speed automatic scanning technology has emerged as a powerful tool for mapping and characterizing electromagnetic fields in various environments. By providing accurate, high-resolution, and spatially-resolved measurements of electromagnetic fields, EMF scanning has found numerous applications in industries such as telecommunications, healthcare, and scientific research.
The principles of EMF scanning, including the use of specialized sensors, scanning techniques, and data processing algorithms, have enabled the development of increasingly sophisticated and capable scanning systems. Moreover, recent advances in EMF scanning technology, such as high-resolution scanning, real-time scanning, multi-physics scanning, and the integration of artificial intelligence and machine learning, are pushing the boundaries of what is possible with this technology.
As the demand for reliable, efficient, and safe electromagnetic systems continues to grow, the importance of EMF scanning in ensuring the compatibility, performance, and safety of these systems cannot be overstated. With ongoing research and development in this field, it is clear that electromagnetic field high speed automatic scanning technology will play an increasingly crucial role in shaping the future of our electromagnetic world.
EMF Scanning Application | Key Benefits |
---|---|
Electromagnetic Compatibility Testing | – Identify sources of interference – Optimize device layout – Ensure compliance with EMC standards |
Wireless Network Optimization | – Map signal coverage and interference – Optimize network node placement – Predict network performance |
Medical Imaging and Diagnostics | – Generate high-resolution images of body tissues – Study brain activity and function – Guide surgical interventions |
Scientific Research | – Investigate electromagnetic phenomena in plasmas, materials, and astrophysical objects – Characterize the electromagnetic properties of materials – Develop new electronic and photonic devices |
Leave a Reply