Understanding Signal Integrity and Power Integrity
Signal integrity (SI) and power integrity (PI) are crucial aspects of high-speed electronic systems. As data rates continue to increase, ensuring the quality and reliability of signals becomes more challenging. This article will delve into the fundamentals of signal and power integrity, discussing key concepts, design considerations, and best practices for achieving optimal performance in high-speed applications.
What is Signal Integrity?
Signal integrity refers to the ability of an electronic system to maintain the quality and fidelity of signals as they propagate through interconnects, such as printed circuit board (PCB) traces, cables, and connectors. The main goal of signal integrity is to ensure that the transmitted signal reaches the receiver with minimal distortion, allowing for reliable communication and data transfer.
Factors Affecting Signal Integrity
Several factors can impact signal integrity in high-speed systems:
-
Reflections: When a signal encounters an impedance discontinuity, such as a trace-to-connector interface, a portion of the signal energy is reflected back to the source. These reflections can cause signal distortion and reduce the signal-to-noise ratio (SNR).
-
Crosstalk: Crosstalk occurs when signals from one trace couple to adjacent traces through capacitive or inductive coupling. This can lead to unwanted noise and interference, compromising signal integrity.
-
Attenuation: As signals travel through interconnects, they experience attenuation due to resistive losses and dielectric absorption. This can result in reduced signal amplitude and increased rise/fall times, affecting the signal’s ability to be correctly interpreted by the receiver.
-
Jitter: Jitter refers to the timing variations of signal transitions. It can be caused by various factors, such as noise, crosstalk, and power supply fluctuations. Excessive jitter can lead to bit errors and reduced system performance.
What is Power Integrity?
Power integrity focuses on ensuring a clean and stable power supply to all components in an electronic system. It involves managing the power distribution network (PDN) to minimize voltage fluctuations, reduce noise, and maintain the required voltage levels for proper device operation.
Factors Affecting Power Integrity
Several factors can impact power integrity in high-speed systems:
-
Voltage Drops: As current flows through the PDN, voltage drops occur due to the resistance of the power and ground planes, as well as the inductance of vias and traces. These voltage drops can cause the supply voltage to deviate from its nominal value, potentially affecting the performance and reliability of the devices.
-
Ground Bounce: Ground bounce occurs when multiple devices switch simultaneously, causing a sudden increase in current demand. This can lead to voltage fluctuations on the ground plane, which can couple to signal traces and introduce noise.
-
Power Supply Noise: Switching noise from voltage regulators, as well as noise coupled from other sources, can propagate through the PDN and affect the power supply quality. This noise can manifest as ripple, spikes, or other unwanted variations in the supply voltage.
-
Electromagnetic Interference (EMI): High-frequency currents flowing through the PDN can radiate electromagnetic energy, causing EMI. This can interfere with other electronic devices and violate electromagnetic compatibility (EMC) regulations.
Designing for Signal Integrity
To ensure signal integrity in high-speed systems, several design techniques and considerations should be taken into account:
Impedance Matching
Matching the impedance of the signal path is crucial for minimizing reflections and ensuring efficient power transfer. This involves designing traces, vias, and connectors to have a consistent characteristic impedance, typically 50 ohms for single-ended signals and 100 ohms for differential pairs.
Termination Strategies
Proper termination techniques help absorb reflections and minimize signal distortion. Common termination strategies include:
-
Series Termination: A resistor is placed in series with the driver to match the impedance of the trace. This helps absorb reflections at the source end.
-
Parallel Termination: A resistor is placed at the receiver end, parallel to the trace. This absorbs reflections at the receiver end and helps maintain signal integrity.
-
AC Termination: Capacitors and resistors are used to provide frequency-dependent termination, which can be effective for high-speed signals with a wide bandwidth.
Signal Routing and Layer Stackup
Proper signal routing and layer stackup are essential for minimizing crosstalk and reducing EMI. Some guidelines include:
-
Minimize Crosstalk: Route sensitive signals away from each other to reduce crosstalk. Use guard traces or ground shields between critical signals to provide additional isolation.
-
Optimize Layer Stackup: Arrange signal layers to minimize the coupling between adjacent traces. Use ground planes between signal layers to provide shielding and reduce crosstalk.
-
Avoid Discontinuities: Minimize vias, stubs, and other discontinuities in the signal path to reduce reflections and signal distortion.
-
Length Matching: Ensure that the lengths of traces within a differential pair or a parallel bus are matched to maintain signal integrity and minimize skew.
Simulation and Analysis
Performing signal integrity simulations and analysis is crucial for identifying potential issues early in the design process. Tools such as SPICE (Simulation Program with Integrated Circuit Emphasis) and electromagnetic field solvers can help predict the behavior of signals and identify areas for improvement.
Designing for Power Integrity
To ensure power integrity in high-speed systems, several design techniques and considerations should be taken into account:
Power Distribution Network (PDN) Design
A well-designed PDN is essential for maintaining a clean and stable power supply. Some key aspects of PDN design include:
-
Low Impedance: Minimize the impedance of the PDN to reduce voltage drops and maintain a stable supply voltage. Use wide power and ground planes, as well as multiple decoupling capacitors, to achieve low impedance.
-
Decoupling Capacitors: Place decoupling capacitors close to the power pins of devices to provide local charge storage and minimize voltage fluctuations. Use a combination of bulk, ceramic, and low-ESL (equivalent series inductance) capacitors to cover a wide frequency range.
-
Power Plane Segmentation: Divide the power plane into separate segments for different voltage domains or noise-sensitive circuits. This helps isolate noise and prevents it from propagating throughout the system.
Voltage Regulator Considerations
Choosing the appropriate voltage regulators and designing their implementation is crucial for power integrity. Consider the following:
-
Regulator Selection: Select voltage regulators with low output ripple, fast transient response, and adequate current capacity to meet the requirements of the system.
-
Regulator Placement: Place voltage regulators close to the devices they supply to minimize the inductance of the power path and improve transient response.
-
Regulator Feedback: Ensure that the feedback loop of the voltage regulator is stable and properly compensated to avoid oscillations and maintain a clean output voltage.
Power Sequencing and Ramp-up
Power sequencing and ramp-up are important considerations, especially in systems with multiple voltage domains or power-sensitive devices. Ensure that the power supplies are turned on in the correct order and with the appropriate ramp-up times to avoid voltage spikes or latch-up conditions.
Simulation and Analysis
Similar to signal integrity, performing power integrity simulations and analysis is essential for identifying potential issues and optimizing the PDN design. Tools such as PDN analyzers and electromagnetic field solvers can help predict voltage drops, impedance profiles, and current density distributions.
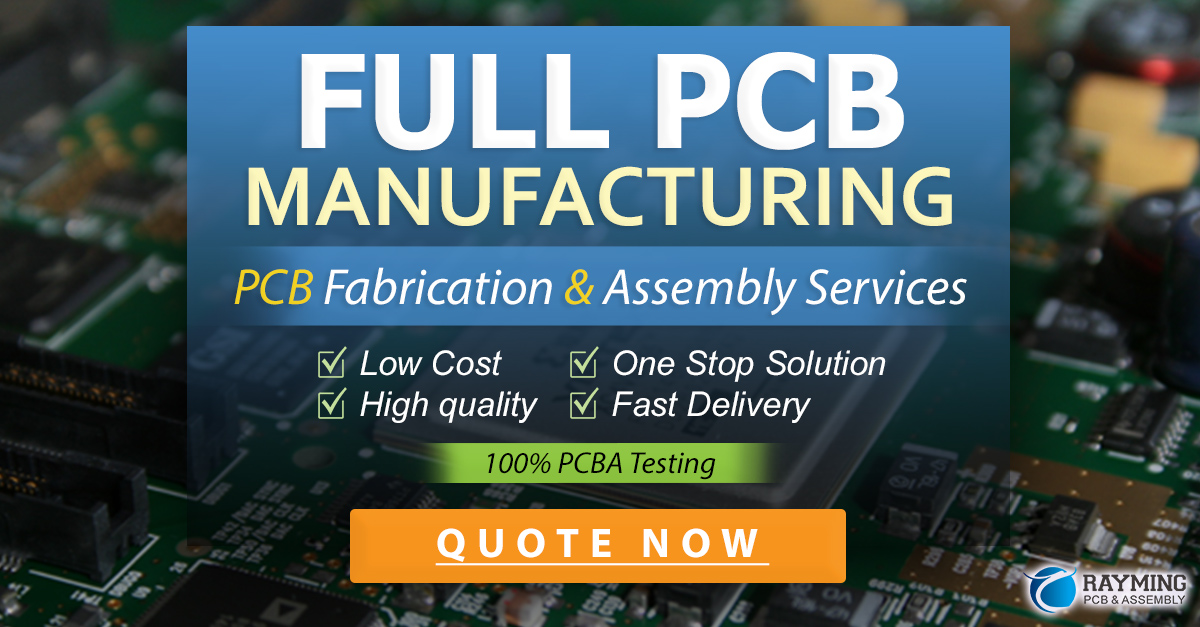
Best Practices for Signal and Power Integrity
To achieve optimal signal and power integrity in high-speed systems, consider the following best practices:
-
Early Planning: Incorporate signal and power integrity considerations from the beginning of the design process. Early planning helps identify potential issues and allows for more effective mitigation strategies.
-
Collaborative Design: Foster collaboration between signal integrity engineers, power integrity engineers, PCB designers, and other stakeholders to ensure a holistic approach to system design.
-
Simulation-Driven Design: Utilize simulation tools extensively to predict and analyze the behavior of signals and power distribution networks. Simulations help identify potential issues and enable proactive design optimization.
-
Design Reviews: Conduct regular design reviews to assess the signal and power integrity aspects of the system. Involve experts from various disciplines to provide comprehensive feedback and catch potential issues early.
-
Testing and Validation: Perform thorough testing and validation of the system to verify signal and power integrity in real-world conditions. Use high-bandwidth oscilloscopes, spectrum analyzers, and other specialized equipment to measure and analyze the system’s performance.
-
Continuous Improvement: Embrace a culture of continuous improvement and learning. Stay updated with the latest techniques, tools, and best practices in signal and power integrity to ensure the design remains robust and reliable.
Frequently Asked Questions (FAQ)
1. What is the difference between signal integrity and power integrity?
Signal integrity focuses on maintaining the quality and fidelity of signals as they propagate through interconnects, while power integrity focuses on ensuring a clean and stable power supply to all components in the system.
2. Why is impedance matching important for signal integrity?
Impedance matching is important for minimizing reflections and ensuring efficient power transfer. When the impedance of the signal path is matched, reflections are minimized, and the signal can propagate with minimal distortion.
3. What are the common termination strategies for signal integrity?
Common termination strategies include series termination (placing a resistor in series with the driver), parallel termination (placing a resistor at the receiver end), and AC termination (using capacitors and resistors for frequency-dependent termination).
4. How do decoupling capacitors help with power integrity?
Decoupling capacitors provide local charge storage and help minimize voltage fluctuations. They are placed close to the power pins of devices to supply the instantaneous current demands and maintain a stable supply voltage.
5. What tools are used for signal and power integrity simulations?
Tools such as SPICE (Simulation Program with Integrated Circuit Emphasis), electromagnetic field solvers, and PDN analyzers are commonly used for signal and power integrity simulations. These tools help predict the behavior of signals and power distribution networks, allowing for proactive design optimization.
Conclusion
Signal and power integrity are critical aspects of high-speed electronic systems. As data rates continue to increase, ensuring the quality and reliability of signals and maintaining a clean and stable power supply become increasingly challenging. By understanding the fundamentals of signal and power integrity, applying appropriate design techniques, and following best practices, designers can mitigate potential issues and achieve optimal system performance.
Key considerations for signal integrity include impedance matching, termination strategies, signal routing, and simulation-driven analysis. For power integrity, designers must focus on PDN design, voltage regulator selection and placement, power sequencing, and ramp-up considerations.
By adopting a collaborative and proactive approach to signal and power integrity, designers can create robust and reliable high-speed systems that meet the ever-increasing demands of modern applications. Continuous learning and staying updated with the latest techniques and tools are essential for staying ahead in this dynamic field.
Aspect | Focus | Key Considerations |
---|---|---|
Signal Integrity | Maintaining signal quality and fidelity | – Impedance matching – Termination strategies – Signal routing – Simulation and analysis |
Power Integrity | Ensuring clean and stable power supply | – PDN design – Voltage regulator selection and placement – Power sequencing and ramp-up – Simulation and analysis |
By addressing both signal and power integrity aspects comprehensively, designers can create high-speed systems that deliver optimal performance, reliability, and robustness in today’s demanding applications.
Leave a Reply