Understanding Signal Integrity and Impedance
Signal integrity is a critical aspect of modern electronic systems, particularly in high-speed digital designs. It refers to the ability of an electrical signal to maintain its quality and accuracy as it propagates through a transmission line or interconnect. One of the key factors that influence signal integrity is characteristic impedance.
What is Characteristic Impedance?
Characteristic impedance, often denoted as Z0, is the ratio of the voltage to the current in a transmission line at any point along its length. It is an intrinsic property of the transmission line and depends on its physical dimensions, materials, and the frequency of the signal being transmitted.
In an ideal transmission line, the characteristic impedance remains constant throughout its length. When a signal encounters a change in impedance, such as a mismatch between the source, transmission line, or load, a portion of the signal is reflected back towards the source. These reflections can lead to signal distortion, overshoot, undershoot, and other signal integrity issues.
Factors Affecting Characteristic Impedance
Several factors influence the characteristic impedance of a transmission line:
-
Geometry: The physical dimensions of the transmission line, such as the width, thickness, and spacing between conductors, play a significant role in determining its characteristic impedance.
-
Dielectric constant: The dielectric constant of the insulating material surrounding the conductors affects the capacitance of the transmission line, which in turn impacts the characteristic impedance.
-
Frequency: The characteristic impedance of a transmission line is frequency-dependent. At higher frequencies, the impedance may deviate from its nominal value due to the skin effect and dielectric losses.
-
Materials: The conductivity and permeability of the materials used for the conductors and the dielectric properties of the insulating materials also contribute to the characteristic impedance.
Impedance Matching and Termination
To ensure good signal integrity, it is essential to match the impedances of the source, transmission line, and load. When impedances are matched, the signal can propagate without reflections, minimizing signal distortion and power loss.
Impedance Matching Techniques
-
Series termination: A resistor is placed in series with the source to match the impedance of the transmission line. This technique is suitable for point-to-point connections.
-
Parallel termination: A resistor is placed in parallel with the load, equal to the characteristic impedance of the transmission line. This method is useful for multi-drop bus configurations.
-
Thevenin termination: A combination of series and parallel resistors is used to match the impedance and provide a stable reference voltage for the signal.
-
AC termination: Capacitors and resistors are used to provide frequency-dependent termination, which is useful for high-speed signals with a wide bandwidth.
Termination Strategies
-
Source termination: The termination resistor is placed near the source to absorb reflections from the load.
-
End termination: The termination resistor is placed at the end of the transmission line, near the load, to prevent reflections from propagating back to the source.
-
Distributed termination: Resistors are placed at regular intervals along the transmission line to absorb reflections and minimize signal distortion.
Signal Integrity Analysis and Simulation
To ensure good signal integrity, designers often rely on analysis and simulation tools to predict the behavior of signals in their systems.
Time-Domain Analysis
Time-domain analysis involves studying the waveforms of signals as they propagate through the system. This analysis helps identify issues such as overshoot, undershoot, ringing, and crosstalk. Tools like oscilloscopes and time-domain reflectometers (TDRs) are used for time-domain measurements.
Frequency-Domain Analysis
Frequency-domain analysis examines the spectral content of signals and the frequency response of the system. It helps identify bandwidth limitations, resonances, and other frequency-dependent effects. Tools like network analyzers and spectrum analyzers are used for frequency-domain measurements.
Electromagnetic Simulation
Electromagnetic simulation tools, such as finite-element analysis (FEA) and method of moments (MoM), are used to model the electromagnetic behavior of transmission lines and interconnects. These tools can predict the characteristic impedance, propagation delay, and coupling between adjacent traces.
circuit Simulation
Circuit simulation tools, such as SPICE (Simulation Program with Integrated Circuit Emphasis), are used to model the electrical behavior of the system, including the effects of terminations, driver and receiver characteristics, and power supply noise. These simulations help designers optimize their designs for signal integrity.
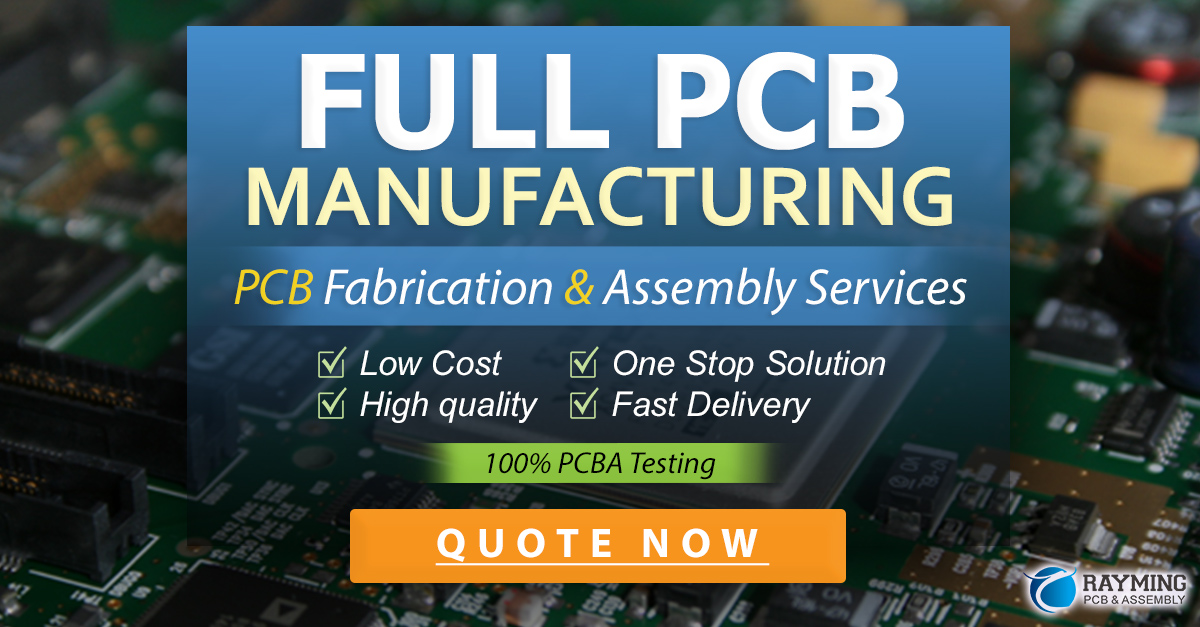
PCB Design Considerations for Signal Integrity
Printed circuit board (PCB) design plays a crucial role in maintaining signal integrity. Here are some key considerations:
Trace Routing
- Keep trace lengths as short as possible to minimize propagation delay and attenuation.
- Avoid sharp bends and corners, which can cause reflections and impedance discontinuities.
- Maintain consistent trace width and spacing to ensure a uniform characteristic impedance.
- Use smooth curves and chamfered corners to minimize reflections.
Layer Stack-up
- Use a balanced and symmetrical layer stack-up to minimize warpage and ensure consistent impedance.
- Provide adequate ground and power planes to minimize inductance and provide a low-impedance return path for signals.
- Use appropriate dielectric materials with stable properties over the frequency range of interest.
Crosstalk Mitigation
- Increase spacing between adjacent traces to reduce crosstalk.
- Use guard traces or ground traces between sensitive signals to provide shielding.
- Minimize parallel run lengths of adjacent traces to reduce coupling.
- Use differential signaling for noise immunity and reduced electromagnetic interference (EMI).
Via Design
- Minimize the number of vias to reduce impedance discontinuities and signal reflections.
- Use appropriate via sizes and pad stack designs to ensure good signal integrity.
- Avoid stubs and unnecessary via-to-via transitions, which can cause resonances and reflections.
Power Distribution Network (PDN)
- Provide a low-impedance power distribution network to minimize power supply noise.
- Use decoupling capacitors near ICs to provide a local reservoir of charge and reduce high-frequency noise.
- Optimize the placement and values of decoupling capacitors based on the frequency range of the signals.
Advanced Topics in Signal Integrity
Equalization Techniques
Equalization techniques are used to compensate for the frequency-dependent losses and dispersion in transmission lines. There are two main types of equalization:
- Pre-emphasis: The signal is pre-distorted at the transmitter to compensate for the expected losses in the transmission line.
- De-emphasis: The signal is attenuated at the receiver to reduce the high-frequency components and minimize inter-symbol interference (ISI).
Jitter Analysis
Jitter refers to the timing variations in a digital signal. It can be caused by various factors, such as noise, crosstalk, and power supply fluctuations. Jitter analysis involves measuring and characterizing jitter to ensure that it remains within acceptable limits for reliable data transmission.
Bit Error Rate (BER) Testing
Bit error rate testing is used to assess the reliability of a digital communication system. It involves sending a known sequence of data through the system and comparing the received data with the original sequence to determine the number of bit errors. BER testing helps identify the impact of signal integrity issues on the overall system performance.
Real-World Applications
Signal integrity and characteristic impedance considerations are crucial in various real-world applications:
-
High-speed digital interfaces: Interfaces like USB, PCIe, SATA, and HDMI require careful design to ensure good signal integrity and minimize reflections.
-
Wireless communications: Antennas and transmission lines in wireless systems must be impedance-matched to maximize power transfer and minimize signal distortion.
-
Automotive electronics: The harsh automotive environment, with its high temperatures, vibrations, and electromagnetic interference, demands robust signal integrity design practices.
-
Aerospace and defense: Reliable communication and data transfer are critical in aerospace and defense applications, where signal integrity is paramount.
-
Medical devices: Signal integrity is essential in medical devices to ensure accurate measurements, reliable data transmission, and patient safety.
Frequently Asked Questions (FAQ)
- What is the difference between characteristic impedance and Input Impedance?
-
Characteristic impedance (Z0) is an intrinsic property of a transmission line, determined by its physical dimensions and materials. It remains constant along the length of the line. Input impedance, on the other hand, is the impedance seen looking into a network or device from a specific point. It depends on the characteristic impedance, the length of the line, and the termination at the far end.
-
How do you measure characteristic impedance?
-
Characteristic impedance can be measured using a time-domain reflectometer (TDR) or a vector network analyzer (VNA). A TDR sends a fast rise-time pulse down the transmission line and measures the reflected signal to determine the impedance profile along the line. A VNA measures the scattering parameters (S-parameters) of the transmission line, from which the characteristic impedance can be derived.
-
What is the effect of impedance mismatch on signal integrity?
-
Impedance mismatch occurs when the impedances of the source, transmission line, and load are not equal. It causes a portion of the signal to be reflected back towards the source, leading to signal distortion, overshoot, undershoot, and ringing. These reflections can degrade signal quality, increase bit error rates, and limit the maximum data rate of the system.
-
How can you minimize crosstalk in PCB designs?
-
Crosstalk can be minimized by increasing the spacing between adjacent traces, using guard traces or ground traces for shielding, minimizing parallel run lengths, and using differential signaling. Other techniques include optimizing the layer stack-up, using stripline or microstrip geometries, and avoiding broadside coupling between layers.
-
What is the role of termination in signal integrity?
- Termination is used to match the impedances of the source, transmission line, and load to minimize reflections and ensure good signal integrity. Proper termination absorbs the reflected energy, preventing it from propagating back to the source and causing signal distortion. Termination techniques include series termination, parallel termination, Thevenin termination, and AC termination, depending on the specific application and signal characteristics.
Conclusion
Signal integrity and characteristic impedance are essential considerations in the design of modern electronic systems. Understanding the factors that affect characteristic impedance, implementing appropriate impedance matching and termination techniques, and following best practices in PCB design are crucial for ensuring reliable and high-quality signal transmission.
As digital systems continue to push the boundaries of speed and complexity, the importance of signal integrity analysis and simulation cannot be overstated. By leveraging advanced tools and techniques, designers can optimize their designs for signal integrity, minimize signal distortion, and ensure the overall performance and reliability of their systems.
In conclusion, a deep understanding of signal integrity and characteristic impedance is essential for engineers and designers working on high-speed digital systems. By applying the principles and techniques discussed in this article, they can effectively tackle the challenges of signal integrity and design robust, reliable, and high-performance electronic systems.
Leave a Reply